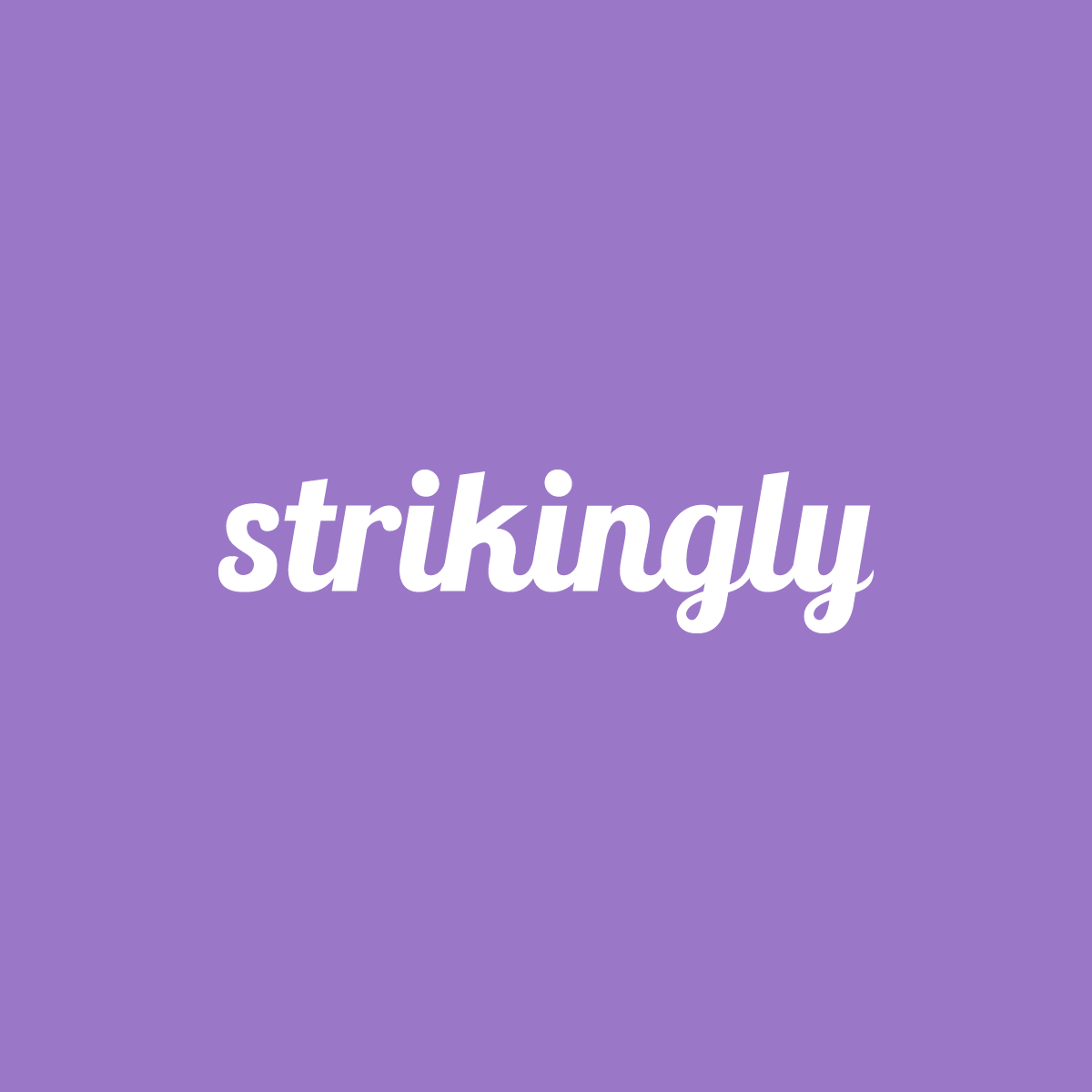
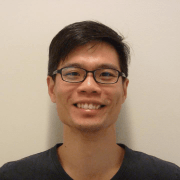
Kuan-Ju Lu
The Graduate Institute of Biotechnology, NCHU, Taichung, Taiwan
Welcome to Kuan-Ju Lu's lab
Researches about the intercellular transport and plant development
Why is intercellular transport important?
The rise of multicellular organisms by delegating different tasks to specific cell types/tissues roots the domination of multicellular organisms around the world. The exchange of information between cells is the core of multicellularity. There are several types of information changes used in all multicellular organisms: receptor-ligand, direct contact, and direct transport. For plant cells, the strong cell wall provides the physical support of vertical growth, however, the thick cell wall also hampered the direct contact and direct transport of molecules between cells. To overcome the difficulty, plants evolved a specialized membrane-based channel, called plasmodesmata (plural form of plasmodesma), that spanned through the cell wall between two cells.
Since the discovery of plasmodesmata (PD) by Dr. Eduard Tangle in the 19th century, scientists are keen to understand what is the significance to have PD and what is the function of plasmodesmata. "PD may involve in transport molecules between cells" seems to be a very logical hypothesis since PD connect two adjacent cells. The observation under an electron microscope (EM) has revealed many details of PD.
What do plasmodesmata do in plants?
The left figure shows that a plasmodesma has a compressed ER tubule, called desmotubule, across neighboring cells. The space between the desmotubule and the surrounding plasma membrane is named cytoplasmic sleeve and is supported by spike proteins (red lines in the above figure). The diameter of a plasmodesma from the perpendicular viewpoint is about 30 - 40 nm and is considered as the passage for passive transport (diffusion) of micro molecules. However, due to the size of the micro molecules, there is no direct observation of a micro-molecule transport through a plasmodesma.
Besides micro molecules, scientists also discovered that macromolecules like proteins and RNAs could be transported through plasmodesmata. In 1990, Dr. David Jackson and Dr. Willam Lucas published that a transcription factor from Maize, named KNOTTED 1 (KN), can dilate the plasmodesmata and carry its own mRNA to travel to adjacent cells at the epidermal cells of a tobacco leaf. In the following three decades, scientists identified more than a dozen proteins, mostly transcription factors, that can move through PD to surrounding cells.
How do plants control intercellular protein transport?
Plants adapt the protein transport mechanism as one of the strategies to determine cell identity and form patterns. The earlier event occurs at the very beginning of the plant life cycle - the embryogenesis. During embryogenesis, auxin and its downstream transcription factor AUXIN RESPONSE FACTOR 5/MONOPTEROS (ARF5/MP) activate a key protein, TARGET OF MONOPTEROS 7 (TMO7), at cells surrounding the hypophysis - the future root apical meristem cell (panel c; asterisk indicates the hypophysis). TMO7 then moves into the target cell to determine its cell fate (panel b). Interestingly, this mobility property is also present in the primary root. The TMO7 promoter expresses majorly in cells surrounding the quiescent center and columella cells (green signal in the pTMO7-3nGFP root). When TMO7-GFP is expressed under its own promoter, the protein moves efficiently into the very tip of the primary root (green signal in the pTMO7-TMO7-GFP root). However, when fusion with a large size 3 time GFP as TMO7-3GFP, the protein becomes stock in cells that expresses the fusion protein (green signal in the pTMO7-TMO7-3GFP).
It has been shown previously, proteins below 60 kDa can transport through PD in the root apical meristemic zone. The fact that TMO7-3GFP proteins are trapped in the originated cells suggests that TMO7 moves from cell to cell through PD. To validate whether TMO7 is transported via PD, we crossed the pTMO7-TMO7-GFP line with a mutant that is known to block PD transport, the CalS3-2d mutant. We observed the decrease of the green signal at the very tip of the root (pTMO7-TMO7-GFP in CalS3-2 in the above figure). These data show that TMO7 transport through plasmodesmata in the mature root tip.
Cis-elements contribute to TMO7's transport
To reveal how TMO7 transport through plasmodesmata, we designed a linker-scanning analysis to replace the original TMO7 amino acids with a series of alanine (m1 - m11). When expressed under the same TMO7 promoter, we clearly observed two major groups of transgenic plants. One has the same transportability as the wild-type TMO7, as TMO7m4 and m7 in the figure. The other group has defective transportability, as TMO7m5, TMO7m8, and TMO7m9 in the figure. This data suggests there is a sequence-dependent mechanism that regulates TMO7 transport.
Exploring the protein transport mechanisms in a non-vascular plant, Marchantia polymorpha
So how exactly plants regulate the intercellular protein transport? This is the question we would like to address in the long-term. To achieve this goal, we designed several strategies to explore the regulation of PD in a non-vascluar model plant, Marchantia polymorpha. Marchantia belongs to the liverwort, together with hornwort and moss are bryophytes. Bryophytes are sister groups of vascular plants and both bryophytes and vascular plants diverged from the most recent comment ancestor to evulve independently for more than 300 million years. Interestingly, most of the key biological processes and components, like plasmodesmata, are preserved in bryophytes and vascular plants, indicating these factors are indispensable for land plants.
Research directions
The long-term goal of our research is to find out how PD were evolved and what is the intercellular protein transport machinery
The origin of plasmodesmata
Investigating potential factors that leads to the evolution of PD
Land plants are considered evolved from algae. The most recent common ancestor (MRCA) of all land plants is believed to be evolved from the filamentous Zygnematophyceae, which have no PD. Therefore, some key factors must have been evolved from Zygnematophyceae to contribute to PD formation. We would like to use the current knowledge from Arabidopsis to dig into the information dataset we can obtain and search for potential keys to PD.
Protein transport in Marchantia polymorpha
Explore the core machinery of protein transport
Bryophytes are non-vascular plants that have diverged from vascular plants about 450 million years ago. Along with the evolution, it seems that PD are crucial for the survival of all land plants therefore none of the land plants has abandoned PD. However, some of the known components to regulate PD permeability cannot be identified in bryophytes like Marchantia polymorpha (a liverwort). It is therefore very interesting to explore how bryophyte regulates the transport of molecules across PD and what are the common components between the PD in Marchantia and in Arabidopsis.
PD composition in Marchantia polymorpha
Looking into common and diverged compositions of PD in sister group species of vascular plants
Another direct way to understand the mechanism of transport via PD is to look into what actually makes a plasmodesma. We designed to isolate PD from Marchantia and compare it with the known Arabidopsis PD proteome to clarify the similarity or divergence between these two species.
Affinity purification of mobile protein interactors
Searching for key factors which control the intercellular protein transport
Tens of thousands of proteins are expressed within a cell, how does a cell distinguish which proteins to transport but not others? One of the hypotheses is proposed, called the ZIP code hypothesis: within mobile proteins, specific protein sequences can be recognized by the transport machinery proteins and deliver the protein to neighboring cells. We will apply a new affinity-based precipitation method to reveal the potential interacting partners of selected mobile proteins.
Publications
Research articles
Evolution of vascular plants through redeployment of ancient developmental regulators. PNAS (2019), 117:733-740. https://www.pnas.org/content/117/1/733.short
Kuan-Ju Lu+, Nicole van ’t Wout Hofland+,Eliana Mor, Sumanth Mutte, Paul Abrahams, Hirotaka Kato, Klaas Vandepoele, Dolf Weijers* and Bert De Rybel*
+Equal contribution
Regulation of intercellular TARGET OF MONOPTEROS 7 protein transport in the Arabidopsis root. Development (2018), 145: dev152892. https://doi.org/10.1242/dev.152892
Kuan-Ju Lu, Bert De Rybel, Hilda van Mourik, Dolf Weijers*
Long-distance movement of Arabidopsis FLOWERING LOCUS T RNA participates in systemic floral regulation. RNA Biology (2012), 9: 653-662.
https://doi.org/10.4161/rna.19965
Lu, K. J., Huang, N. C., Liu, Y. S., Lu, C. A., and Yu, T. S.*
Review articles
Peeking at a plant through the holes in the wall – exploring the roles of plasmodesmata. New Phytologist (2018), 218: 1310-1314.
https://doi.org/10.1111/nph.15130
Kuan‐Ju Lu*, Florence R. Danila, Yueh Cho, Christine Faulkner.
Building a plant: cell fate specification in the early Arabidopsis embryo. Development (2014), 142: 420-430. https://doi.org/10.1242/dev.111500
ten Hove, C.A., Lu, K. J. and Weijers D.*
Peoples
Kuan-Ju Lu
Apr. 2016 - Oct. 2019. Postdoctoral research fellow VIB Center for Plant Systems Biology, Belgium, and Wageningen University and Research center, the Netherlands.
Apr. 2014 - Mar. 2016. Postdoctoral research fellow Wageningen University and Research center, the Netherlands.
Sep. 2007 - Jun. 2012. Ph.D. in Biotechnology, Molecular and Biological Agricultural Sciences (MBAS) Program, Taiwan International Graduate Program (TIGP), National Chung-Hsing University.查看更多詳情...
© 2019